Learning Objectives
- Differentiate between phenotypic expression and gene expression
- Recognize and explain examples of quantitative traits, multiple allelism, polygenic inheritance, gene-by-gene interactions, and gene-by-environment interactions
- Explain incomplete and co-dominance, predict phenotypic ratios for incomplete and co-dominance, and use genotypic and phenotypic ratios to determine if traits are incomplete or co-dominant
- Recognize that traits with dominant/recessive and simple Mendelian patterns of inheritance (e.g., 3:1, 9:3:3:1) are rare, and that traits are complex, meaning they are influenced by multiple genes and non-genetic effects
- Differentiate among patterns of inheritance influenced by one, two, or more than two genes
A note about the word “expression”
The word “expression” can mean different things in different contexts. In molecular biology, “expression” means “transcribed and translated,” or the process of making a protein from the genetic instructions in DNA.
In discussions of phenotypes, sometimes people use the word “expressed” to mean “visible” in the phenotype.
These very different definitions create a lot of confusion about the difference between gene expression and phenotypic appearance, because it can make it sounds like a recessive allele is recessive because it must not be transcribed or translated. This is not the case. Often both the dominant and the recessive alleles are expressed (transcribed and translated), but the behavior of the protein encoded by the dominant allele “masks” or “hides” the behavior of the protein encoded by the recessive allele.
Recognizing this distinction is extremely helpful for understanding the behavior of both Mendelian (single gene, dominant/recessive inheritance) and “non-Mendelian” traits (anything other than single gene, dominant/recessive inheritance).
Beyond dominant/recessive traits
Mendel identified the rules of particulate inheritance (inheritance based on genes) using pea plants which have many single-gene traits with a dominant/recessive inheritance pattern. This is the simplest inheritance pattern possible, and most traits are NOT controlled this way. Other (more common) inheritance patterns include:
- Incomplete dominance: where heterozygotes have an intermediate phenotype in-between the two homozygous phenotypes. An example is petal color in four-o’clock flowers, where homozygotes are either white or red, and heterozygotes are pink. Each R allele contributes one ‘unit’ of petal color, while each r allele contributes no ‘units’ of petal color. So two R alleles results in a red, one R allele results in pink, and no R alleles results in white.
- Co-dominance: where heterozygotes display each phenotype associated with each allele. An example is AB blood type in humans, where the A allele results in one specific type of sugar on a red blood cell, and B results in a different type of sugar on a red blood cell. Two A alleles results in only A-type sugars, two B alleles results in only B-type sugars, and the heterozygote has both A- and B-type sugars on the red blood cell. (Type O results in no sugar; we’ll discuss this more in class.) Though they seem similar at first glance, incomplete dominance and co-dominance are different from each other, and are based on the molecular phenomenon underlying the trait.
Here is a video describing the difference between incomplete dominance and co-dominance using an artificial example
- Quantitative traits: where the trait has a continuous phenotype controlled by additive alleles at multiple genes. This means that the trait is not controlled by just one gene with several alleles, but MULTIPLE genes (polygenic inheritance), each of which can have multiple alleles. An example is human height: we have differences in height down to fractions of an inch, rather than being either 4 ft, 5 ft, or 6ft tall. Each height allele at each gene controlling height contributes a ‘unit’ of height which is additive. Quantitative traits are in contrast to discrete traits where the trait has only a few possible phenotypes which fall into discrete classes (ie, peas are either round or wrinkly, and there are no in-between phenotypes).
- Multiple allelism: where a particular gene has more than two alleles circulating in the population. An example is human blood type (described above) where the single gene controlling blood type can be have an A, B, or O allele.
This video describes the difference between polygenic traits and multiple allelism:
And this video works through some real examples of multiple allelism and quantitative traits (stop at 6:20 min):
- Gene-by-gene interactions: where the phenotype associated with one allele depends on the allele(s) present at another gene. This is different from a quantitative trait where alleles at multiple genes are additive. The gene-by-gene inheritance pattern can also be called epistasis. The take home-message on gene-by-gene interactions is that this phenomenon alters the expected phenotypic ratios of a Mendelian dihybrid cross (9:3:3:1) to a different pattern.
This video gives an overview of a gene-by-gene interaction that controls coat color in mice:
- Pleiotropy is the phenomenon where a single gene influences multiple, seemingly unrelated traits. For example, in the human disorder phenylketonuria (PKU), a single mutation in a single gene can cause intellectual disability, seizures, reduced skin pigmentation, light hair color, “musty” smelling urine, and a predisposition to eczema.
- Gene by environment interactions: where the environment plays a role in determining phenotype controlled by alleles. An example is human height (which is also an example of a quantitative trait) where childhood nutrition plays a role in an adult height. We have gotten taller as a species in the last 200 years (mostly) not because of changes in our alleles but due to access to better nutrition in much of the world.
While these types of inheritance ‘violate’ Mendel’s rules for inheritance of single-gene discrete traits, they are all still controlled by the behavior of chromosomes during meiosis. In addition, the single-gene inheritance pattern Mendel discovered is actually pretty rare compared to all these other inheritance patterns described above: most traits are controlled by one or more of the inheritance patterns described above. In class, we’ll predict genotypes, phenotypes, and phenotypic ratios for incomplete dominance and co-dominance inheritance patterns.
Sustainable Development Goal
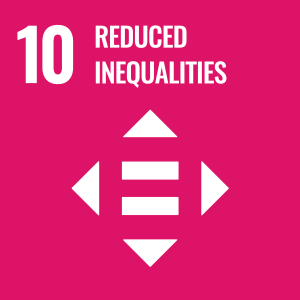
UN Sustainable Development Goal (SDG) 10: Reduced Inequalities – Recognizing that genes act in concert with other genes and the environment to determine traits can help reduce inequalities in healthcare and education by promoting more personalized and targeted approaches. Understanding the genetic basis for differences in traits and diseases can help reduce inequalities in healthcare and access to resources.
A great example of gene by environment interactions is the tyrosinase mutation that causes the distinctive color patterning of Siamese cats.
http://www.brighthub.com/science/genetics/articles/40501.aspx