Learning Objectives
- Describe the interactions between DNA and proteins that regulate transcription initiation in prokaryotes and eukaryotes
- Differentiate between constitutive and regulated promoters
- Distinguish positive regulation from negative regulation, and compare and contrast mechanisms for each in prokaryotes and eukaryotes
- Explain the roles of chromatin and alternative splicing in eukaryotic gene regulation
- Compare and contrast mechanisms for gene co-regulation in prokaryotes and eukaryotes
- Use a model gene regulatory system to predict the effects of various mutations or malfunctions on gene expression
Overview of Gene Regulation
Cells express (transcribe and translate) only a subset of their genes. Cells respond to environmental signals by turning on or off expression of appropriate genes. In multicellular organisms, cells in different tissues and organs differentiate, or become specialized by making different sets of proteins, even though all cells in the body (with a couple of exceptions) have the same genome. Such changes in gene expression, or differential gene expression among cells, are most often regulated at the level of transcription.
There are three broad levels of regulation of gene expression:
- transcriptional control (whether and how much a gene is transcribed into mRNA)
- translational control (whether and how much an mRNA is translated into protein)
- post-translational control (whether the protein is in an active or inactive form, and whether the protein is stable or degraded)
Based on our shared evolutionary origin, there are many similarities in the ways that prokaryotes and eukaryotes regulate gene expression. There are also many differences. All three domains of life use positive regulation (turning on gene expression), negative regulation (turning off gene expression), and co-regulation (turning multiple genes on or off together) to control gene expression, but there are some differences in the specifics of how these jobs are carried out between prokaryotes and eukaryotes.
Similarities between prokaryotes and eukaryotes: promoters and regulatory elements for transcription initiation
Promoters are sites in the DNA where RNA polymerase binds to initiate transcription. Promoters also contain, or have near them, binding sites for transcription factors, which are DNA-binding proteins that can either help recruit, or repel, RNA polymerase. A regulatory element is a DNA sequence that certain transcription factors recognize and bind to in order to recruit or repel RNA polymerase. The promoter and the nearby transcription factor binding elements together regulate gene transcription.

Regulatory elements can be used for either positive or negative transcriptional control. When a gene is subject to positive transcriptional control, the binding of a specific transcription factor to the regulatory element promotes transcription. Alternatively with negative transcriptional control, the transcription factor binds to a regulatory element to repress transcription. A single gene can be subject to both positive and negative transcriptional control by different transcription factors, creating multiple layers of regulation.
But some genes are not subject to regulation. These genes are constitutive, or constitutively expressed, meaning they are always transcribed. What sorts of genes would you imagine a cell would always need to have “on,” regardless of the environment or situation?
Additional complexities specific to eukaryotic gene regulation: chromatin and alternative splicing
Another major difference between prokaryotic gene regulation and eukaryotic gene regulation is that the eukaryotic (but not prokaryotic) DNA double helix is organized around proteins called histones that organize the DNA into nucleosomes. This combination of DNA + histones is called chromatin.
Chromatin can be condensed in a 30-nm fiber formation (tightly compacted nucleosomes) or more loosely bound so the DNA between and around nucleosomes is more accessible to transcription factors and RNA polymerases. The degree of compaction is controlled by post-translational modifications that are added to the histones in the nucleosomes. When histones have acetyl groups added to them by enzymes called histone acetyl transferases (HATs), the acetyl groups physically obstruct the nucleosomes from packing too densely and help to recruit other enzymes that further open the chromatin structure. Conversely, when the acetyl groups are removed by histone deacetylases (HDACs), the chromatin assumes a condensed formation that prevents transcription factors from being able to access the DNA. In the image below, you can clearly see how much more compact and inaccessible the 30-nm fiber is (top) compared to the more loosely wound formation (bottom).
Chromatin plays a fundamental role in positive and negative gene regulation because transcriptional activators and RNA polymerase cannot physically access the DNA regulatory elements when chromatin is in a compact form.
Prokaryotic DNA does have some associated proteins that help to organize the genomes, but it is fundamentally different from chromatin. Prokaryotic DNA can essentially be thought of as ‘naked’ compared to eukaryotic chromatin, so prokaryotic cells lack this layer of gene regulation.
Another difference between prokaryotic and eukaryotic gene regulation is that eukaryotic mRNAs must be properly processed with addition of the 5′ cap, splicing out of introns, and addition of the 3′ poly(A) tail (discussed in more detail here). Each of these processing steps is also subject to regulation, and the mRNA will be degraded if any of the steps are not properly completed. The export of mRNAs from the nucleus to the cytoplasm is also regulated, as is stability of the properly processed mRNA in the cytoplasm.
Finally, eukaryotic genes often have different splice variants, where different exons can be included in different mRNAs that are transcribed from the same gene. Below is a cartoon depiction of a gene with color-coded exons showing two different mRNA molecules transcribed from the same gene. The different mRNAs encode for different proteins because they contain different exons. This process is called alternative splicing, and we will discuss it more here.
Often different types of cells in different tissues express different splice variants of the same gene, such that there is a heart-specific transcript and a kidney-specific transcript of a particular gene.
In general, eukaryotic gene regulation is more complex than prokaryotic gene regulation. The upstream regulatory regions of eukaryotic genes have binding sites for multiple transcription factors, both positive regulators and negative regulators, that work in combination to determine the level of transcription. Binding sites for activators are places for positive regulation, while binding sites for repressors generate negative regulation when bound. Some regulatory elements have specific names that describe their roles in gene expression. Two are called enhancers and silencers; they work at quite a distance, thousands of base pairs away from the promoter.
Differences between prokaryotes and eukaryotes: mechanisms of co-regulation
Often a set of proteins are needed together to respond to a certain stimulus or carry out a certain function (for example, metabolizing a specific sugar). There are often mechanisms to co-regulate genes so they are all transcribed in response to the same stimulus. Both prokaryotic and eukaryotic cells have ways of co-regulating genes, but they use very different mechanisms to accomplish .
In prokaryotes, co-regulated genes are typically organized into an operon, where two or more functionally related genes are transcribed together from a single promoter into one long mRNA. This mRNA is translated to make all of the proteins encoded by the genes in the operon. Ribosomes start at the 5′ end, begin translating at the first AUG codon, terminate when they run into a stop codon, and then re-initiate at the next AUG codon.
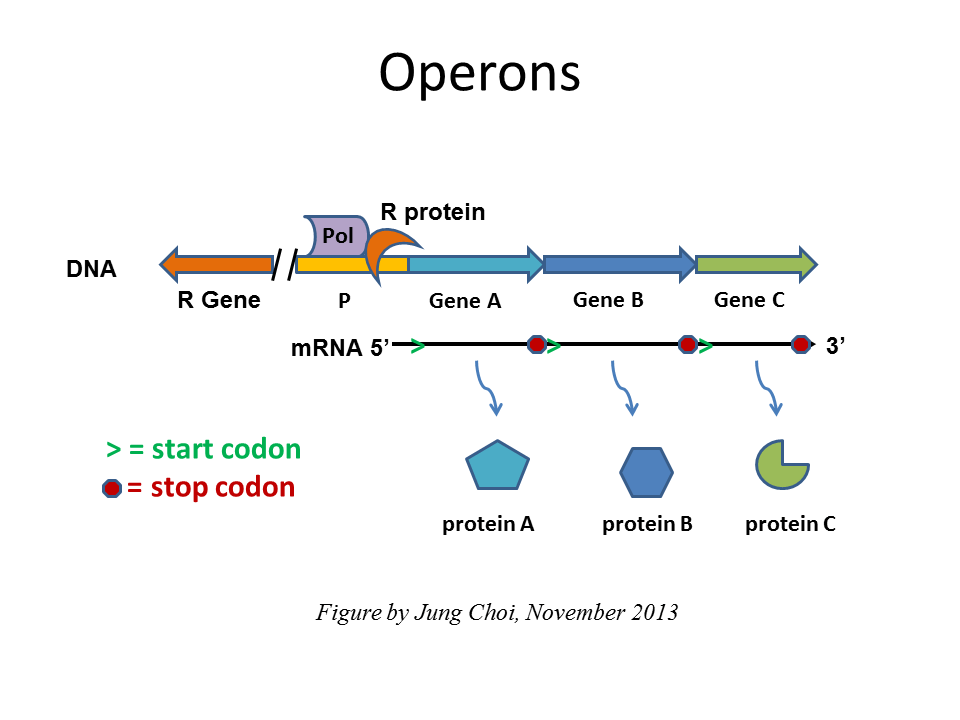
With a few exceptions (C. elegans and related nematodes), eukaryotic genomes do not have genes arranged in operons. Instead, eukaryotic genes that are co-regulated tend to have the same DNA regulatory element sequence associated with each gene, even if those genes are located on completely different chromosomes. This means that the same transcriptional activator or repressor can regulate transcription of every single gene that has that particular DNA regulatory element associated with it. For example, eukaryotic HSP (heat shock protein) genes are located on different chromosomes. HSPs help cells survive and recover from heat shock (a type of cellular stress). All HSP genes are transcribed simultaneously in response to heat stress because they all have a matching DNA sequence element that binds a heat shock response transcription factor.
Overall differences and similarities
If you understand the similarities and differences in eukaryotic and prokaryotic gene regulation, then you know which of the following processes are exclusive to eukaryotes, which are exclusive to prokaryotes, which occur in both, and how each is accomplished:
- coupled transcription and translation
- 5′ cap and 3′ poly(A) tail
- AUG as the translation initiation codon
- regulation of gene expression by proteins binding to DNA regulatory elements
- alternative mRNA splicing
- regulation of gene expression through chromatin accessibility
An example of gene regulation: the lac operon in E. coli
The discovery of the lac operon in 1956 opened up our understanding of gene regulation. It now serves as a solid model of gene regulation in prokaryotes. As you read the information below, make sure you can apply as many details of gene regulation described above to this specific example as you can. For those you cannot apply, consider whether those are specific to eukaryotes.
E. coli lac operon: dual positive and negative regulation

The lac operon of E. coli has 3 structural genes required for metabolism of lactose, a disaccharide found at high levels in milk:
- lacZ encodes the enzyme beta-galactosidase, which cleaves lactose into glucose and galactose
- lacY encodes permease, a membrane protein for facilitated diffusion of lactose into the cell
- lacA encodes transacetylase, an enzyme that modifies lactose
An mRNA encoding all 3 proteins is transcribed at high levels only when lactose is present, and glucose is absent.
Negative regulation by the Repressor—In the absence of lactose, the lac Repressor protein, encoded by the lacI gene with a separate promoter that is always active, binds to the Operator sequence in the DNA. The Operator sequence is a type of DNA regulatory element as described above. Repressor protein bound to the Operator prevents RNA polymerase from initiating transcription.
When lactose is present, an inducer molecule derived from lactose binds allosterically to the Repressor and causes the Repressor to leave the Operator site, unblocking the operon’s promotor. RNA polymerase is then free to initiate transcription, if it successfully binds to the lac promoter.
Positive regulation by CAP—Glucose is the preferred substrate for energy metabolism. When glucose is present, cells transcribe the lac operon only at very low levels, so the cells obtain most of their energy from glucose metabolism. RNA polymerase by itself binds rather poorly to the lac promoter.
Glucose starvation causes a rise in the level of cyclic adenosine monophosphate (cAMP), an intracellular alarm signal. Cyclic AMP binds to the catabolite activator protein (CAP). The CAP+cAMP complex binds to the CAP binding site near the lac promoter and recruits RNA polymerase to the promoter.
High level transcription of the lac operon requires both that CAP+cAMP be bound to the CAP binding site, and that Repressor is absent from the Operator. These conditions normally occur only in the absence of glucose and presence of lactose.
The lac operon in E. coli is a classic example of a prokaryotic operon that is subject to both positive and negative regulation. Positive regulation and negative regulation are universal themes for gene regulation in both prokaryotes and eukaryotes.
Sustainable Development Goal
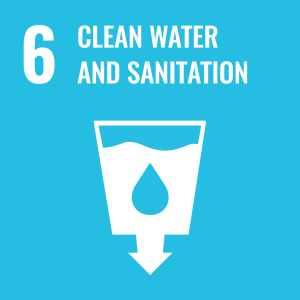
UN Sustainable Development Goal (SDG) 6: Clean Water and Sanitation – Not everyone has easy access to clean water. Research to change this aims to ensure access to clean water and sanitation for all by better understanding the bacteria that live in ‘unclean’ water. Research on gene regulation in microorganisms has lead to the development of new technologies for water purification and wastewater treatment, particularly those that are cheap, effective, and can work in more remote areas of the world.